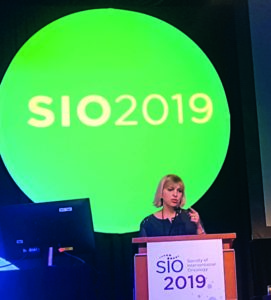
“Interventional radiology is uniquely equipped to shape the next generation of nanotherapeutics,” Sarah White, associate professor of Radiation and Surgical Oncology at the Medical College of Wisconsin (Milwaukee, USA) and adjunct professor of Radiology at Northwestern University (Evanston, USA) informed delegates at the 2019 annual meeting of the Society of Interventional Oncology (SIO; 7–11 June, Boston, USA). She presented research detailing how the local delivery of nanoparticles directly to the tumour site enables enhanced care to cancer patients. nanotechnology
“I think nanotechnology really allows us to troubleshoot some of the problems in interventional oncology, and then to find applications and create solutions”, she said.
Globally, US$10 billion is spent on nanotechnology research, across many different disciplines. Explaining why she believes this technology has so much potential in the medical field specifically, White said, “Nanoparticles are the right size. One nanoparticle is a billionth of a metre. To visualise that: a marble to the size of the Earth. The diameter of a DNA double helix is 2nm, and the smallest bacteria is 200nm long. Nanotechnology is the size between cells and their organelles, such as mitochondria. They are right in that cellular realm, which is why it is so advantageous to use them in this field.”
In cancer research, nanoparticles are usually used in a theranostic setting, meaning that the nanoparticle can be used not only as a drug—as the treatment—but also as an imaging tool. Currently, systemic chemotherapy used to treat cancer patients is non-specific and, due to lymphatic drainage of the chemotherapy agent from the target tissue, needs to be administered in such high doses to be concentrated enough in the tumour to destroy the cancer that side effects are common and severe.
However, when the chemotherapy drugs are formulated into a nanoparticle, these do not leach out of the tumour cells as easily. This is because healthy cells are packed close together in an orderly structure, and have tight junctions with a lymphatic outflow system threaded throughout. In comparison, tumour cells have antigenic factors that mean they grow rapidly, proliferating in a disordered fashion without tight junctions. The larger gaps between tumour cells means the normal lymphatic outflow cannot form to drain the tissue correctly, altering what passes into and out of the cancerous cells. Smaller molecules, such as nanoparticles, can enter the tumour, and due to the lack of lymphatic drainage, remain there. This method of drug delivery is known as passive tumour targeting, and it increases the ability of the interventionist to get the drug into the tumorous tissue. Due to the proper lymphatic drainage of the non-tumour cells in the body, the nanoparticles are filtered out of these more effectively, meaning the concentration of these small molecules is greater in the cancer.
Active targeting of the tumour
Active targeting of the tumour by nanoparticles also improves clinical outcomes. White explained at SIO: “Tumours often overexpress receptors, so we can put things on the nanoparticles to actively target those surplus receptors.”
According to White, this active tumour targeting increases the efficacy of the treatment due to greater internalisation of the chemotherapy agent. Another drug delivery method explained by White at SIO is endothelial cell targeting, where, similarly to the overexpression of surface receptors on the tumour cells, investigators can formulate nanoparticles to specifically target overexpressed receptors on angiogenic endothelial cells. These line the newer blood vessels feeding the tumour, and using nanoparticles to target these cells helps eradicate the source of the blood flow to cancerous or benign growths.
“The last thing we can do [with drug delivery] is triggered drug release”, White described, “and this is one of the most interesting to me. We can stimulate the release of the cargo. The cargo is the imaging agent or the treatment.”
Clinical applications of nanotechnology in interventional oncology
Addressing the SIO audience, White described current and potential clinical applications of nanoparticles. The first example she used was in the delivery of hydrophobic drugs: “We want to deliver hydrophobic drugs. We think sorafenib can help us treat the surge in vascular endothelial growth factor receptor (VEGF) after transarterial chemoembolization (TACE) that we see at 24 hours.”
Sorafenib is currently the only first-line multi-kinase inhibitor approved for use in hepatocellular carcinoma (HCC). It inhibits the activity of several kinases, including VEGF, which are involved in tumour cell signalling, proliferation, angiogenesis and apoptosis. Thus, sorafenib inhibits tumour growth and neoangiogenesis. Several recent studies, including work by Baosheng Ren (The Second People’s Hospital of Changzhou, Changzhou, China), Wansheng Wang (The First Affiliated Hospital of Soochow University, Suzhou, China) et al in the Journal of Cancer, have reported that overall survival is improved in HCC patients when treated with a combination of sorafenib and TACE compared with TACE alone.
“But when sorafenib is administered systemically”, White explained, “which is done in order to get high enough levels of the drug into the tumour, it can cause extreme side effects.”
In an effort to eliminate these side effects, nanoparticles can be used to deliver sorafenib locally, rather than systemically. According to White, it is possible to make a nanoconstruct with sorafenib that will deliver and deposit the drug straight to the tumour. White and collaborators have fabricated poly(lactide-co-glycolide) microspheres for local sorafenib delivery to tumours during liver-directed embolotherapies. These constructs co-encapsulated iron oxide nanoparticles (IONP) so that the investigators could observe microsphere delivery via magnetic resonance imaging (MRI). Following intra-arterial microsphere infusions in a rabbit VX2 liver model, White demonstrated in 2015 that these sorafenib particles normalised VEGF activity and decreased microvessel density. Writing at the time in Biomaterials, she concluded “These methods should avoid systemic toxicities, with MRI permitting follow-up confirmation of microsphere delivery to the targeted liver tumors”.
A second clinical application of nanoparticles in interventional oncology is to expand the ablation zone when treating patients with metastatic colon cancer who are not candidates for resection. The ablation zone is limited by size (4mm being approximately the largest possible size) and location (due to the risk of damage to adjacent structures). Using a rat model of colorectal liver metastases, White and colleagues compared the size of ablation zones in three groups—rats injected with hybrid magnetic gold nanoparticles tagged with monoclonal antibodies, rats injected with hybrid magnetic gold nanoparticles only, and a control group.
After undergoing photothermal ablation with a fibre-coupled near infrared diode laser, haematoxylin-eosin staining revealed a significant difference in tumour necrosis across the three groups. In the antibody and nanoparticles group, tumour necrosis was 38%±29. In contrast, tumour necrosis in the nanoparticles only group was 14%±17, demonstrating a lack of toxicity. The control group, which were only injected with the colorectal liver metastasis cell lines, had a tumour necrosis of 7%±8 (p=0.043). The addition of the monoclonal antibodies to the nanoparticles also resulted in statistically significant higher levels of delivery to the tumour compared to nanoparticles alone. Published in Radiology in 2017, the investigators concluded that targeted monoclonal antibody–conjugated hybrid magnetic gold nanoparticles can serve as a catalyst for photothermal ablation of colorectal liver metastases by increasing ablation zones. nanotechnology